Elucidating the Elusive: Nonlinear Optics Tracks Antiferromagnetism in Real Space
A research collaboration between the Laboratory for Multifunctional Ferroic Materials (ETH Zurich) and the Satoh Lab (Tokyo Institute of Technology) tracks the “invisible” magnetism in an antiferromagnetic material on the move. Understanding the dynamics of antiferromagnets is crucial for the development of electronic devices that are orders of magnitude faster than the existing ones.
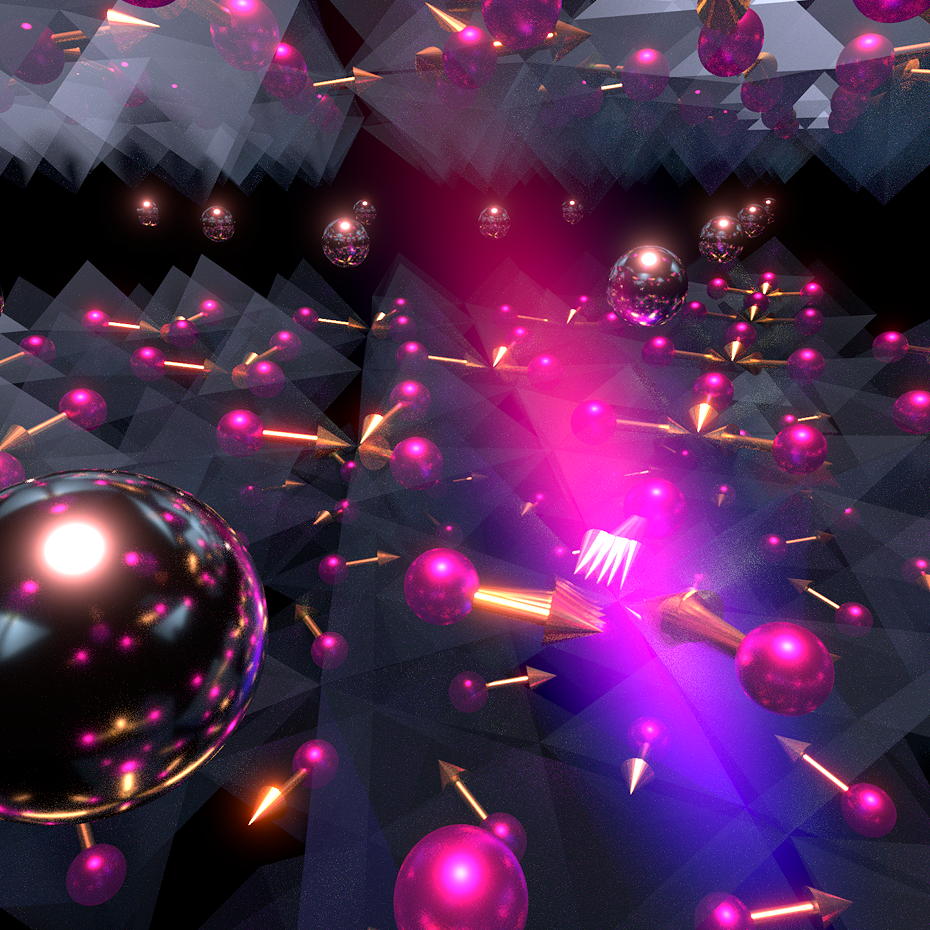
Exciting light: ultrafast optical laser pulses are able to induce coherent spin motions in hexagonal YMnO3. Researchers now showed that optical second harmonic generation in combination with Faraday rotation measurements allows tracking that motion with sub-picosecond time resolution.
Ferromagnets, such as iron, are omnipresent in our everyday life. A typical example is a fridge magnet. Its macroscopic magnetization allows the magnet to stick seemingly effortlessly to the refrigerator door. The same principle laid the foundation for current information technology, which now relies heavily on closely stacked, nanosized magnets to store logical bits in hard drives. When the north poles (or south poles) of two magnets approach each other, however, they experience a repulsive force. The same repulsive force destabilizes bits in hard drives and increases the energy costs of their writing process.
A lesser known, but actually more common form of magnetic order is antiferromagnetism. In contrast to ferromagnetism, antiferromagnetic materials lack a macroscopic magnetization. Antiferromagnets thus avoid that repulsive force. Accordingly, changing from ferromagnetic to antiferromagnetic bits could drastically improve the energy efficiency and data density of magnetic memories, while at the same time enabling switching rates that are orders of magnitude faster.
On the other hand, the absence of magnetization, that is, its “magnetic invisibility”, renders the detection of the antiferromagnetic order challenging. In order to understand and optimize how an antiferromagnet switches, a time-resolved visualization of the antiferromagnetic order is even required. In a proof-of-concept demonstration, researchers were now able to track the full three-dimensional motion of the antiferromagnetic state. By combining linear and nonlinear optical techniques, all components of the vector representing the antiferromagnetic order became optically accessible with sub-picosecond time resolution.
In contrast to the almost circular motion that is expected for ferromagnets, the authors found that the tip of that vector follows a strongly elliptical path, where the ratio of the long axis to the short axis is more than 100. Such a pronounced anisotropy has important implications for the energy-efficient realization of antiferromagnetic switching.
Details of this study are presented in a recent publication in external page Nature Communications
Christian Tzschaschel, Takuya Satoh, Manfred Fiebig: “Tracking the ultrafast motion of an antiferromagnetic order parameter”, Nature Communications (2019). external page DOI:10.1038/s41467-019-11961-9