Inducing Magnetism in Nonmagnetic Metals
Only a few pure elements present a stable magnetization at room temperature (Fe, Co, Ni). Most metals, however, are nonmagnetic. Yet, it is still possible to induce a finite magnetization in otherwise nonmagnetic metals by virtue of a rather exotic phenomenon called the "spin Hall effect". A team of the Magnetism and Interfaces group has succeeded to directly measure the current-induced spin accumulation in bare Pt and W conductors using a very sensitive Kerr effect microscope.
Only a few pure elements present a stable magnetization at room temperature, the best known examples being the three ferromagnetic metals Fe, Co, and Ni. Most metals, however, are nonmagnetic. Yet, it is still possible to induce a finite magnetization in otherwise nonmagnetic metals by virtue of a rather exotic phenomenon called the “spin Hall effect”, first predicted in 1971 by Mikhail Dyakonov and Vladimir Perel of the Ioffe Physical Technical Institute in Leningrad [1].
This phenomenon is named in analogy to the well-known Hall effect, which causes the deviation of the charge carrier flow in a conductor orthogonal to the direction of the primary current, under the influence of an external magnetic field. Unlike the ordinary Hall effect, however, the spin Hall effect is related to the relativistic coupling of the electron's spin and orbital angular momentum and does not require a magnetic field. Simply explained, electrons flowing forward with their spin magnetic moment pointing up, are deviated, say, to the right, whereas spin-down electrons are deviated to the left. Likewise, electrons with their spin pointing left are deviated up, and electrons with their spin pointing right are deviated down. The curved trajectories of the electrons, similar to those of a spinning ball on a football field, result in the accumulation of opposite spins at the opposite edges of a conductor. Because there are an equal number of spin up and spin down electrons in a nonmagnetic metal, no charge imbalance and therefore no transverse voltage is created, which makes this process rather difficult to detect.
It is perhaps for this reason that the spin Hall effect did not attract much interest initially, and remained undetected for over 30 years. The situation changed as the interest in controlling spins by electrical means raised alongside with the prospects of applications in metal and semiconductor spintronic devices. In 2004, Kato et al. managed to probe the spin accumulation induced by the spin Hall effect at the edges of a semiconducting GaAs wire using the magneto-optical Kerr effect [2]. Since then, several schemes have been devised to detect and exploit the spin Hall effect in a variety of materials and devices, including magnetic switches and logic gates [3]. Despite such a flourishing activity, direct measurements of the spin Hall effect in metals have remained elusive. Because of the much faster spin relaxation in metals compared to semiconductors, the region over which the spin accumulate in a metal is only a few nanometers thick, which makes the total current-induced magnetization very small. Now, a team of the Magnetism and Interfaces group at the Department of Materials at ETH has succeeded to directly measure the current-induced spin accumulation in bare Pt and W conductors using a very sensitive Kerr effect microscope [4]. By focusing a laser beam down to a micron spot and increasing and modulating the current density responsible for the spin Hall effect while carefully eliminating thermally-induced changes of the optical reflectivity, the team led by PhD student Christoph Murer, Dr. Christian Stamm, and Prof. Pietro Gambardella managed to detect a tiny Kerr rotation signal, equivalent to about half a millionth of a degree, which is directly proportional to the current-induced magnetization in the metal (Figs. 1-3).
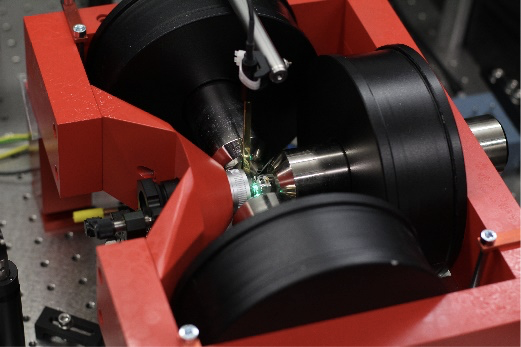
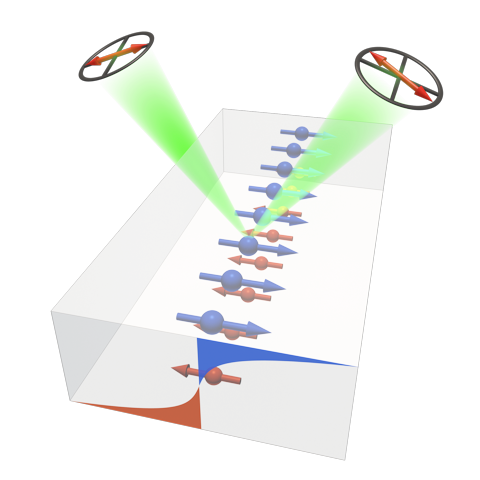
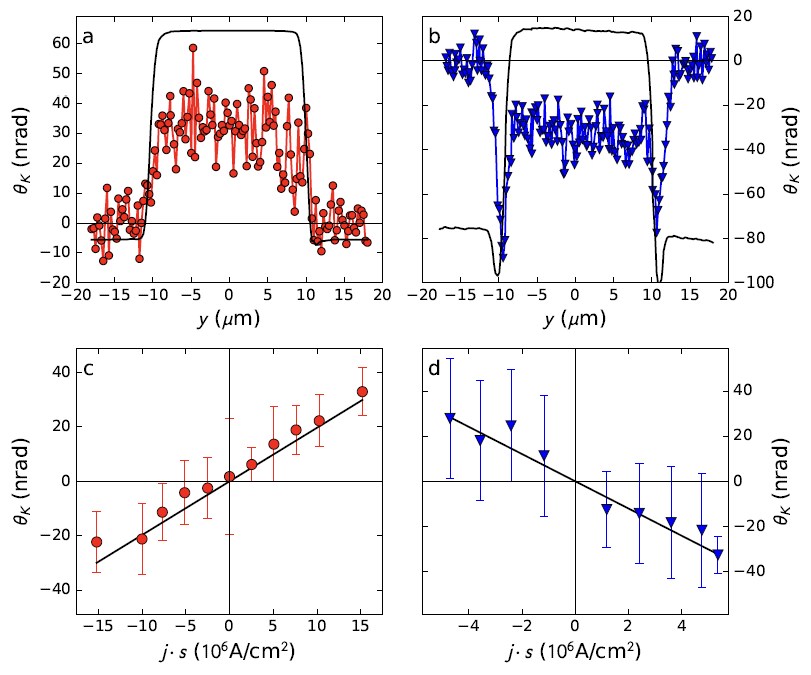
By comparing the experimental data with ab initio calculations of the magneto-optical Kerr effect performed by Dr. Marco Berritta and Prof. Peter Oppeneer at the University of Uppsala, Sweden, the team could quantitatively determine the current-induced spin accumulation at the Pt interface as 2 · 10-5 Bohr magnetons for a current density of 1 · 107 Acm-2. To give an idea, this magnetization is almost 100 000 times smaller than that of Fe! From thickness-dependent measurements, the researchers also determined the spin Hall angle, namely the fraction of the charge current that is converted into a spin current, which amounts to about 8 %, as well as the spin diffusion length of Pt, which is 11±3 nm. Although the induced magnetization is small, the charge-spin conversion rate is actually quite large, meaning that Pt is a strong spin current source that can be used to control the magnetization of ferromagnetic and antiferromagnetic layers placed next to it. This is an active line of investigation worldwide. The quantitative measurements of the current-induced spin accumulation in bare nonmagnetic metals demonstrated here shed light on the strength of the spin Hall effect and open the way to map the spatial and temporal evolution of the spin accumulation and diffusive dynamics in materials with strong spin-orbit coupling.
- M. D’yakonov and V. Perel’, JETP Lett. 13, 467 (1971).
- Y. K. Kato, R. C. Myers, A. C. Gossard, and D. D.Awschalom, Science 306, 1910 (2004).
- J. Sinova, S. O. Valenzuela, J. Wunderlich, C. H. Back, and T. Jungwirth, Rev. Mod. Phys. 87, 1213 (2015).
- Magneto-Optical Detection of the Spin Hall Effect in Pt and W Thin Films, C. Stamm, C. Murer, M. Berritta, J. Feng, M. Gabureac, P. M. Oppeneer, and P. Gambardella, Phys. Rev. Lett 119, 087203 (2017). external page DOI:10.1103/PhysRevLett.119.087203